A couple of weeks ago I posted a photo of Jacqueline Cochran to my Facebook page, along with a note explaining that she was the first woman to break the sound barrier when she flew an F-86 Sabre past the Mach in 1953. A friend posted this comment:
You should add “in level flight”. Lots of WWII fighter pilots broke the sound barrier in combat dives, many died because they did not understand why their controls suddenly became useless.
I responded with this:
There’s a certain amount of mythology about WWII-era prop fighters exceeding the speed of sound in dives. Not known to have ever happened. The control problems some pilots reported happened in transonic flight as shock waves built up around the airplane and the elevators lost authority (which is why supersonic aircraft have all-moving horizontal stabilizers today). The F-86 Cochrane flew was a hopped up Canadian built version with a big engine, and in fact she had to dive to hit the Mach … the F-86 couldn’t go supersonic in level flight. Additional info here.
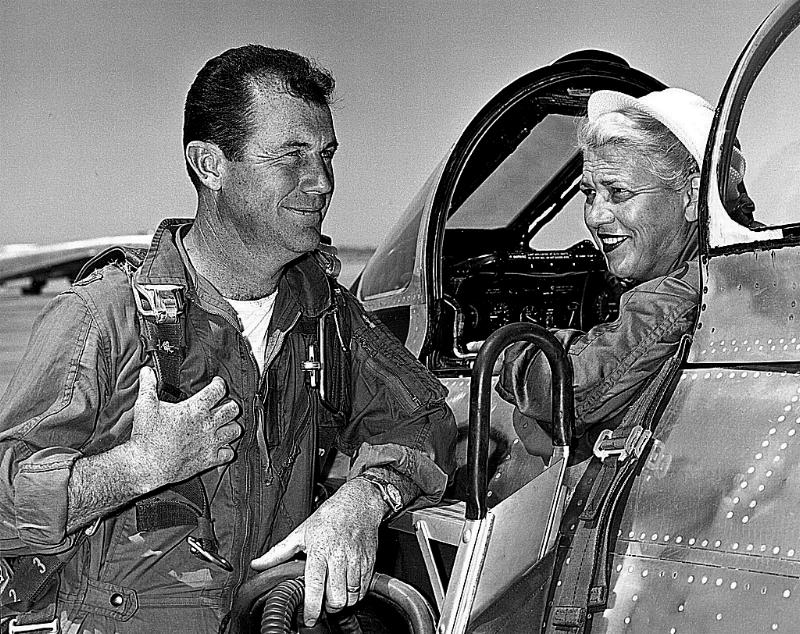
I want to flesh out my response, mostly by way of rumor control. First of all, let me stress my lack of aeronautical engineering credentials. I’m an English major. There’s a lot I don’t understand about the mechanics of transonic and supersonic flight. What I do know is what I was taught in flight school, and what I experienced in my years flying supersonic trainers and fighters. If I screw up some of the principles and technical language, I hope you’ll forgive me, but I think I’m qualified to address some of the apocryphal tales handed down from generation to generation, and to provide a simplified overview of transonic & supersonic flight and the changes to aircraft flight controls necessary to achieve it.
WWII and prop fighters. At the pinnacle of prop fighter development, aircraft like the Lockheed P-38 Lightning and Supermarine Spitfire could attain transonic speeds in steep dives. The transonic range is generally considered to be between Mach 0.8 and 1.0 (600 to 768 mph at sea level, but since mph numbers go down as you climb into thinner air and lower temperatures they’re not really meaningful, so from now on I’ll only talk Mach numbers). Even if we had at the time understood supersonic flight and knew how to build the thin wings and area-ruled fuselages needed for supersonic flight, compressibility would have still made it impossible to force the big disc of a spinning propeller much beyond Mach 0.9.
In one test flight, a Spitfire managed to hit Mach 0.92 in a 45-degree dive, at which point the propeller and reduction gear left for parts unknown. The test pilot was lucky to survive. Other pilot reports from the time stated that it was extremely difficult to recover from transonic dives. Some pilots described the problem as “control reversal,” but that’s not what it was (no one ever had to push forward on the stick to recover from a dive). Control problems resulted from a combination of two factors: one, the physical force required to move the stick aft in a transonic dive was enormous; two, the aircraft’s ailerons and elevators were rendered ineffective by transonic shockwaves forming around the wings and tailplanes. In other words, it was extremely difficult to move the stick in the first place, and when the pilot was able to move it, the ailerons and elevators couldn’t “bite” enough air to control aircraft attitude. The only way to safely recover from a transonic dive was to throttle back to idle and let aerodynamic drag slow the aircraft to a speed at which control effectiveness was regained. Some WWII prop fighters (the P-38 Lightning, for example) actually had speedbrakes that auto-deployed in high speed dives, precisely to keep pilots out of this “coffin corner.”
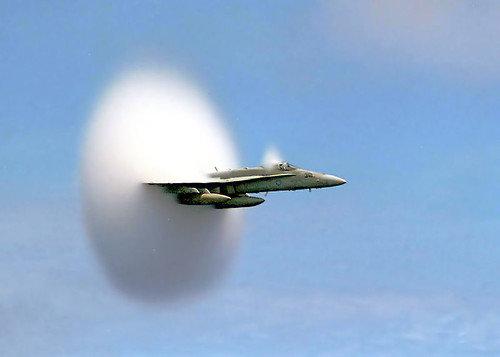
Early jet & rocket fighters. There are unconfirmed stories of Luftwaffe pilots reaching the Mach in the German Me-262, the first jet fighter. Some claim German test pilots went supersonic in prototype rocket-propelled fighters. The stories about the Me-262 breaking the sound barrier, even in a dive, are almost certainly mistaken. The Me-262’s fuselage design and fat wings would be considered incompatible with supersonic flight today. Cockpit airspeed indications sometimes jump around in transonic flight as shock waves form near the pitot tube, and this may have led some pilots to believe they’d exceeded the Mach. The secret weapon rocket planes may well have done it, but if it happened it was never documented.
Some still believe that in 1946, British test pilot Geoffrey de Havilland, Jr. exceeded the speed of sound in an early jet, the de Havilland Swallow, but then encountered control reversal and died in the ensuing crash. Actually, transonic shock waves caused the Swallow’s wings to flutter during a transonic dive and they broke off. That’s what killed Geoffrey de Havilland, but the incident helped create the myth that the speed of sound was an actual “barrier.”
On October 14, 1947, USAF pilot Chuck Yeager achieved true supersonic flight in the rocket-powered Bell X-1 (the design of which, apparently, was originally British). According to an often-repeated story, another test pilot had already gone supersonic in an XP-86 Sabre, but he did it in a dive and his speed wasn’t officially confirmed or recorded, so, as with the Luftwaffe rocket pilots, his achievement didn’t count. Yeager’s speed run was measured and recorded, and he did it in level flight to boot, and that’s the flight that’s in the history books.
I want to go back to flight controls now, the real subject of this post. WWII prop fighters and most early jet fighters had conventional flight controls. The stick and rudder pedals were directly connected to the ailerons, rudder, and elevators by rods, cables, and pulleys. The elevators, which control pitch, were hinged surfaces on the rear of the horizontal tail. When you got into a transonic dive, it became physically difficult to move the elevators, and even when you did, they didn’t have enough authority to bring the nose up and break the dive. In the early jet days, when aircraft like the F-86 Sabre and MiG-15 could get well into the transonic region in level flight, you had the same problems plus a shock wave effect called Mach tuck, which forced your nose down and put you into a dive. You didn’t have enough elevator authority to overcome Mach tuck and stay level. The Soviet MiG-15 had auto-speedbrakes that deployed at Mach 0.92 to keep pilots from exceeding that speed.
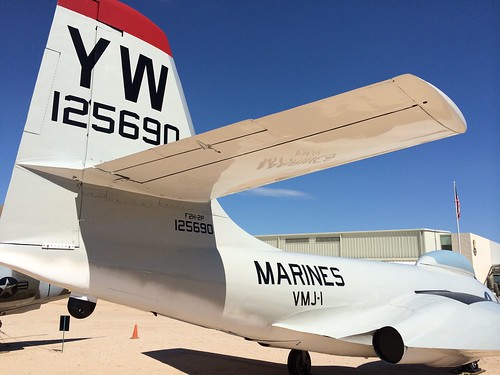
Aircraft designers had been aware of transonic flight control problems from the early days of WWII and had tried several approaches to overcome it. The answer proved to be hydraulically-actuated flight controls and an all-moving tail. With hydraulic assist, pilots no longer had to physically overcome the enormous airflow pressures on their elevators and ailerons. Ditching the hinged elevators and going to all-moving horizontal stabilators gave fast jets enough pitch control to maintain level flight or pull out of a dive as the aircraft began to generate transonic shock waves (side note: once you’re past the Mach the shock wave is behind your aircraft, and a simple hinged elevator would be more than enough to control pitch at supersonic speed … you need the all-moving tail to maintain control as you move through the Mach).
A potential problem with hydraulically-actuated flight controls was that the pilot wouldn’t be able to feel the actual force of the air on the external flight controls. Without that feel, it would be easy to over-control an aircraft at transonic speeds, potentially to the point of ripping the tail off. The solution was to add “artificial feel” to the stick, with the force required to move it increasing it as aircraft speed increased (but nowhere near the force that would be required to move the stick with conventional flight control systems).
The F-86 Sabre, America’s first swept-wing fighter, had hydraulically-actuated flight controls and artificial feel. They say one of the factors that gave our F-86 pilots an edge over the Russian, Chinese, and North Korean MiG-15 pilots they flew against during the Korean War was that our jets weren’t physically exhausting to fly. The MiG, with conventional muscle-powered flight controls, took a lot out of its pilots at speeds above Mach 0.8; moreover, it was dangerously uncontrollable above Mach 0.92 (hence the auto-speedbrakes that kept it from flying faster). In addition to hydraulic assist, later models of the F-86 had all-moving tails. Our guys didn’t have to worry about flying too fast because they could maintain control at all speeds. The MiG bubbas had to constantly watch their speed lest they fly into an uncontrollable flight regime.
The F-86 Sabre is what drew me into this discussion in the first place. When I read that Jacqueline Cochran broke the sound barrier in an F-86, my first thought was “no way” … the Sabre wasn’t supersonic. It had relatively thin wings, but the fuselage wasn’t area ruled, it didn’t have an afterburner, and it was slower than the subsonic MiG-15s it faced in combat in Korea. But as I looked into the subject, it became apparent that the F-86, along with most early swept-wing fighters, could bust the Mach in a dive, and in fact that’s how Jackie did it. The jet she flew was a Canadair Sabre with all-moving horizontal stabilators. As mentioned above, while the first Sabres had conventional tails with hinged elevators, the F-86E and later models had all-moving horizontal stabs.
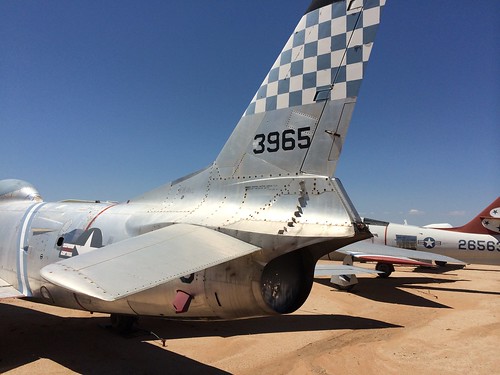
What did I do next? I took photos of the tail sections of two late-model F-86 Sabres on display at the Pima Air & Space Museum, where I’m a volunteer docent. The F-86L, pictured above, had the single-piece all-moving horizontal stabs I expected to see, but the F-86H sitting next to it appeared to have conventional horizontal stabilizers with hinged elevators, and that I did not expect to see. The F-86H was the last, and hottest, F-86 model built, and according to everything I’d read it was supposed to have all-moving horizontal stabs. Here’s what I saw when I looked at the F-86H:
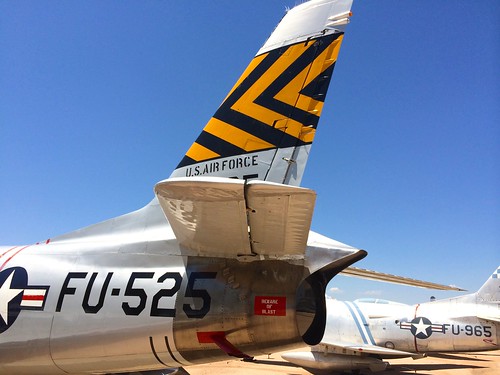
Certainly looks conventional, doesn’t it? I started asking around, hoping one of the other volunteers might know someone who had flown Sabres. Two weeks later, my tree-shaking paid off: I heard from a pilot who’d flown every model of the F-86. Here’s what he said:
The F-86A had conventional tail controls: a fixed stabilizer with an attached moveable elevator which was moved either by the pilot’s control stick or by an electric trim motor. This design proved to be largely ineffective in the supersonic regime (above .9 Mach) where recovery from a supersonic dive required very large angles of elevator movement which exerted so much stress that it sometimes caused rivets to pop out from the trailing edge. Pilots complained that the flight controls appeared to be “strange” in the transonic speed range. They seemed to be “reversed” — if the pilot wanted to pull up and his speed was near Mach 1, the aircraft continued to go down. The controls did not actually reverse, they simply did not respond effectively.
The F-86E tail was called an “all-flying tail”. The F-86E’s elevators and horizontal stabilizer operated as one unit. The horizontal stabilizer was pivoted at its rear spar so that the leading edge was moved eight degrees up or down by the normal action of the control stick. The elevator was mechanically linked to the stabilizer and moved in a specific relation to the stabilizer movement, with the elevator travel being slighter greater than stabilizer travel. This effectively created a larger elevator surface — as the pilot called for more elevator, the stabilizer would move in conjunction with the elevator, creating a greater angle of attack, thus giving better control at all speeds. The all-flying tail of the F-86E eliminated many of the undesirable compressibility effects that were characteristic of the F-86A. It made recovery from a sonic dive much more straightforward, with much less danger of structural damage or catastrophic failure. Externally, the only difference between and F-86A and E was the presence of a bulge in the fuselage of the E immediately in front of the stabilizer to cover the gearing mechanism.
The picture … of your F-86H shows it to have the same all-flying tail as was on the E and F models. It would work in the same manner as I have described above.
All-moving horizontal stabilators are standard equipment on supersonic fighters and trainers today. You’ll also see all-moving stabilators on many airliners, which cruise in the Mach 0.8-0.9 regime. On modern fighters, the stabilators work differentially to control roll as well as pitch. Here are the stabs on the fighter I flew, the F-15 Eagle:
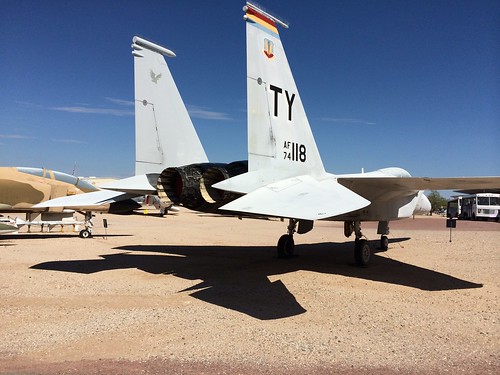
What you don’t see very often is an all-moving vertical tail. Yaw isn’t a big deal in transonic flight, not like pitch, so a conventional hinged rudder on a fixed vertical tailplane works well. The F-15, if you stomped in full right or left rudder, gave you 30 degrees of deflection. Above Mach 1.5, however, a mechanical limiter kicked in and you could only move the rudders 5 degrees. This was one of the things I had to check during the supersonic portion of functional check flights, and I always fed in rudder slowly, visions of snapped off vertical tails prompting caution. Nevertheless a few supersonic aircraft had all-moving vertical tails, presumably with limiters similar to those on the F-15. A few I can think of off-hand were the SR-71, XB-70, F-107, and the A-5 Vigilante:
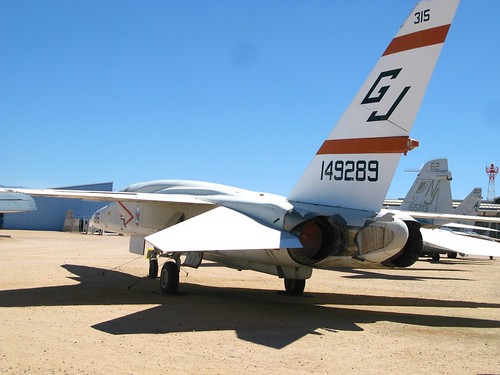
The forces acting on an aircraft and its flight control surfaces at transonic speeds are enormous. Flying at those speeds in early jet fighters was a much bigger deal than it is today, and I can’t tell you what it was like. I can tell you what it’s like in an F-15 Eagle, though.
The first few times I went through the Mach I wasn’t attuned to my environment, but the more experience I gained the more I could sense my speed through the feel of the aircraft. When the Eagle got up to around Mach 0.95 you’d begin to feel resistance, as if the air was getting thicker and pushing back against your airplane. As you slipped past the shockwave and through the Mach the resistance went away and the airplane felt normal again. The second you retarded the throttles, though, it was as though you’d run into an invisible Stay-Puft Marshmallow Man in the air. You and your jet and everything in it slowed down in a hurry, forcing you forward against the shoulder straps and seat belt. Once you were back below Mach 0.95, Mr. Stay-Puft went away and things felt normal again. Oh, and half your fuel was gone.
No one asked, but my personal speed record is Mach 2.21, achieved in a clean F-15C on a functional check flight over the North Sea. On paper the Eagle is capable of Mach 2.5, but I’ve never heard of anyone reaching that speed.
I’m only going to say this once, not because you, dear reader, don’t know it, but you’d be surprised how often people ask me, and who knows, one of them may read this some day: yes, you can hear yourself talk when you’re supersonic; no, you can’t hear the sonic boom.
Well, that was a whole bunch of nerdy tl;dr, all generated by a comment to a one-paragraph Facebook post commemorating Jackie Cochran’s supersonic flight in 1953. I know I’m leaving a lot out, but I wanted to hit the important parts. Next time you hear people telling tall tales about supersonic Mustangs and Thunderbolts, their brave pilots heroically overcoming every natural instinct in the face of control reversal, perhaps you can point them my way. Or at least pass on the link to this post.
Unbelievably informative, thank you. Strange that people would ask if you could hear yourself talk when you were supersonic . . . why not? But I am still a little unclear on the F-86H and the picture of its tail. So even though it is clearly segmented, it moved as one unit?
James, if you look at the F-86H tail photo in full size on Flickr, you can see the single pivot point for the stab, pretty much right in the middle where it meets the aft fuselage. The entire stabilator moves around that pivot as one unit, but the “elevator” part can move another couple degrees if the pilot pulls all the way back on the stick. As far as I know, this “two-piece-acting-as-one-piece” configuration is unique to the F-86E and later Sabres. All the other jets, once we went to all-moving tails, used one-piece stabs.
Appreciate the response!
I cross-posted this entry to Daily Kos, where a reader left the following comment, full of clarifying and interesting information:
(1) The photo of the F-18 “shock wave” is a photo of a vapor cone aka ‘Prandtl-Glauert singularity”Prandtl-Glauert singularity’. It’s a low pressure zone behind the building shockwave, made visible in the photo by the condensation of water — lower pressure air can hold less water than high-pressure air. This can also be seen in less extreme circumstances over the wings of planes turning or pulling high-g maneuvers at airshows — fog forms over the wings near the fuselage as the plane turns or pulls hard. They’re regularly seen at airshows when it’s humid or cloudy out. They’re also often seen behind airliner wing tips as they descend out of clouds.
(2) The speed of sound is almost entirely determined by temperature, and very little by pressure. Air gets colder as one gets further from the Earth, however, so the speed of sound drops with that until the stratosphere, which has more-or-less constant temps, is reached.
(3) The ‘coffin corner’ has different meanings in different contexts. In general, subsonic aircraft have a few numbers of merit. One is Vne (“never exceed” speed), which is typically a true airspeed, i.e., the actual speed of the aircraft through the air. (More formally, Vne is often a ‘mixed airspeed’ phenomena, depending on a number of parameters, but it’s usually treated as a true airspeed.)
Another is the stall speed of an aircraft, and the stall speed is generally an indicated airspeed — a function of how much air the wings “feel”. The true stall speed of an aircraft increases substantially with the thinning of the air.
At some altitude (the “absolute ceiling”), these speeds meet — Vne, which does not change with altitude, and stall speed, which increases with altitude.
The danger/problem is that near the absolute ceiling, where the two speeds match, recovering from a stall is impossible — the nose drops in stall, speeding the aircraft up, putting it past Vne, where it may break up in flight.
Similar problems occur for aircraft such as the U-2, where stall and mach-buffet may occur within a few knots of each other, and supersonic aircraft, where there are max-Q (aerodynamic loading) restrictions.
(4) Aircraft control during supersonic flight does have issues of its own beyond those of compressibility. One is that a wing’s center of lift retreats substantially (moves backwards) at supersonic speeds, tending to pitch the nose down (this is the major component of “mach tuck”). Considerable control authority is required, perhaps well beyond that that might be encountered in subsonic flight regimes.
(5) The ‘control reversal’ problem can certainly be an issue with subsonic aircraft. As mentioned, this can be due to stiffness issues — e.g., the B-52 does not use ailerons for roll control. An aileron on the B-52’s long, thin, floppy wings would tend to twist the wing, increasing or decreasing the lift on that wing more than the aileron would make up for. Instead spoilers are used to decrease lift on either wing to roll the aircraft.
(6) Flutter, aka “undamped aeroelastic vibration” can, depending on the aircraft, occur at speeds well below the transonic regime. Again, this is typically a problem with aircraft stiffness, particularly the ‘twistiness’ of the main wings and tail surfaces, and also typically involves the weighting of the control system + aerodynamic surfaces: ailerons and other control surfaces often have mass balances, to minimize the tendency of the ailerons to move when the whole wing is subject to an abrupt g-load. They are also usually made deliberately stiff in torsion around the aircraft pitch axis.
For subsonic aircraft, flutter is often the primary limitation on Vne. Google has some impressive videos of flutter (an aircraft and the Tacoma Narrows bridge); it is capable of shaking an airplane to destruction in a few seconds.
(7) As mentioned, the transonic regime is high-drag. Aircraft that regularly cruise at supersonic speeds (e.g., SST, SR-71) typically do a ‘dipsy doodle’ to minimize their time at transonic speeds. In the SR-71, this was reportedly done between 30,000′ and 35,000′ or so by climbing to something below 35,000′ at subsonic speed, diving to reach a supersonic speed, and a supersonic climbout to cruise altitude.
Hi Paul. I have a metal model of an F-15, signed by Gen Yeager along w picture of him signing model letter of authenticity. Treasured memento.
Also since you spoke of F-86 in this article, my dad was AF pilot who started on the 86!
I’m a retired airline, US Airways, pilot and my brother is a Captain for Delta.
Thanks for you article!
Great post. As you note, ASI fluctuations at high speed convinced WWII flyers that some had exceeded sonic but that really was not possible with those aircraft. The F-86 though was certainly flown through the ‘barrier’ by George Welch some time before the official record breaking flight. Almost nobody adds the obligatory ‘in level flight’ when writing about the X-1, 2nd aircraft to ‘break the sound barrier’ flown by the 2nd pilot to do so. Now I hope I don’t get sued by anyone from Oroville, CA.
That hinged part of the all-flying F-86H tailplane might be a trim tab.
‘Tod’ recently posted…ERCO Ercoupe Light Sport Aircraft: Antique Flying Art